Lightwave valleytronics in a monolayer of tungsten diselenide
F. Langer, C.P. Schmid, S. Schlauderer, M. Gmitra, J. Fabian, P. Nagler, C. Schüller, T. Korn, P.G. Hawkins, J.T. Steiner, U. Huttner, S.W. Koch, M. Kira, R. Huber
Nature 557 (2018) 76
The next step in modern information technology, so-called quantum information technology, are robust and quickly switchable electronic states provided by “valley pseudospin” which can be changed when energized electrons are optically driven between distinct energetic valleys in hexagonal layered materials. Taking only a few femtoseconds to achieve and in room temperature air, “lightwave valleytronics” may take efficient storage, processing and communication beyond “Moore’s law”.
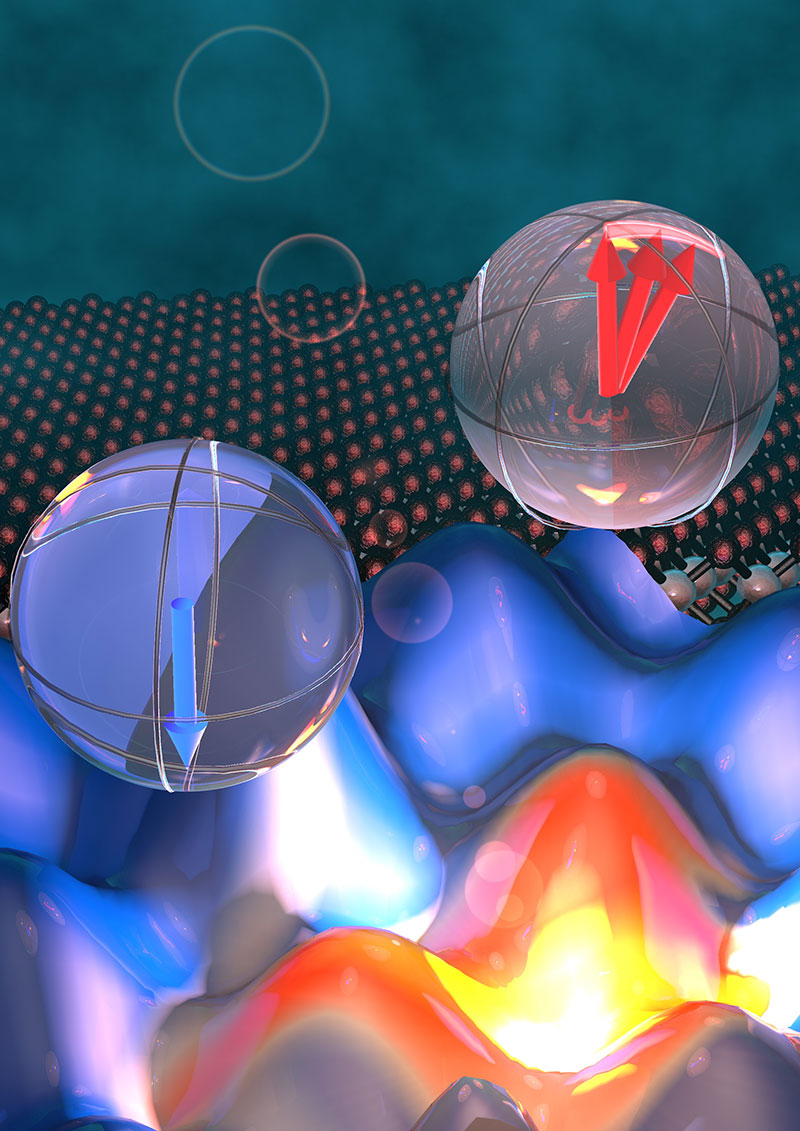
Energy-landscape in a WSe2-semiconductor depicted as blue hills. Electrons are accelerated from one valley to the next (yelloworange) with the colored arrows indicating change of the valley-pseudospin.
As a consequence of the hexagonal bandstructure in TMDCs, novel quantum aspects materialize as two different energy valleys appear at the K and K’ points of the Brillouin zone. Whether an electron resides in K or K’ valley or a superposition of both can be described by a spin-like quantity, the valley pseudospin. Electrons can be selectively excited in either of these valleys by using right- or left-circular polarized light. The aspiration to develop pseudospin-qubit operations (qubits can be mixtures, i.e. superpositions, of the 1 or 0 states) has prompted a novel research field dubbed “valleytronics”.
Using a combination of optimized optical and THz excitation pulses, the record-fast valley pseudospin switching is demonstrated in a monolayer of WSe2. In the experiments, a strong terahertz light pulse accelerates the previously photo-generated charge carriers. As predicted by fully microscopic calculations, the flipping qubit was directly observed as changed circular polarization of light emitted by the electrons. More specifically, the experiment–theory team from Marburg, Regensburg and Michigan found that after excitation with circularly polarized light, the emission contained distinct opposite circular polarization due to the pseudo-spin flip. While the current experiment transfers 66% of valley-polarized electrons to the other valley, theory shows how a fidelity of 96% can be reached with slightly improved parameters, paving the way for developing room-temperature quantum signal processing.
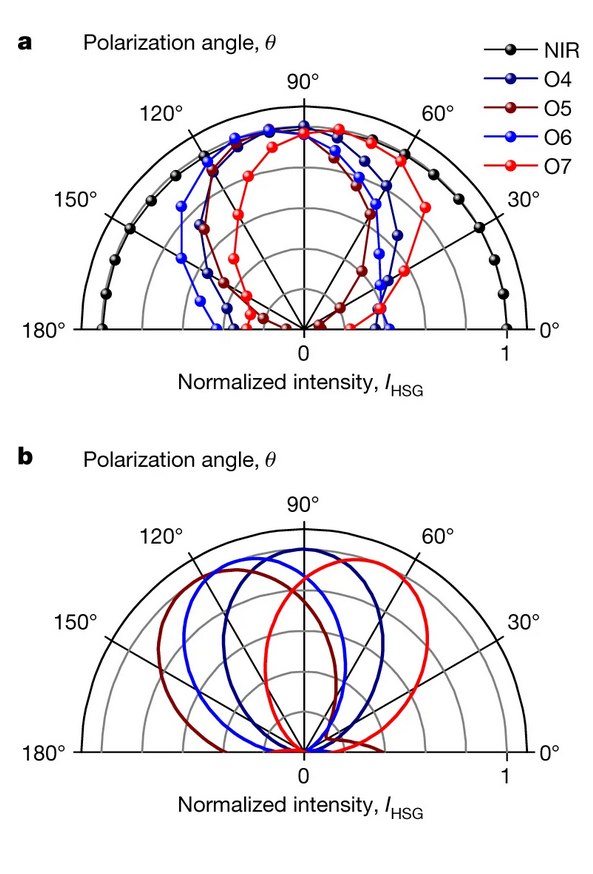
(a) Measured polarization-resolved intensity IHSG of individual sideband orders (normalized, coloured data points) for circularly polarized excitation (normalized, black data points) of the K valley only. (b) Computed polarization of high-order sidebands (orders four to seven, solid lines, same colours as in a) following a valley-selective excitation in the K valley.